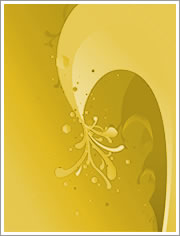
Current Personnel:
- Yong Sup Ihn
- Hector Chen
Former Personnel:
- Melanie Kittle
- Laura Feeney
- Tarn Burton
- Brian Derickson
Current Personnel:
Former Personnel:
As far as we know, the standard model of elementary particle physics accounts for all known physical phenomena. Well-motivated experiments to search for phenomena that cannot be described by the standard model are therefore crucial to advancing our understanding of nature at its most basic level. We are carrying out one such experiment, in which we are searching for an intrinsic electron electric dipole moment (edm). Such a moment is forbidden to exist at observable levels within the standard model, but is allowed in many extensions of the standard model at the level of 10-26 e·cm. This lies very near the current experimental bound. Our experiment will look for this electron edm by looking for shifts in magnetic resonance lines of laser-cooled Cs atoms in an electric field. The idea behind the experiment is that if the electron has a dipole moment, the atom has one also. The atomic levels would then shift due to the first order interaction between the atomic dipole moment and the electric field. Our approach will be to hold the atoms in an optical dipole force trap. The perturbations to the atomic levels due to the trap can be made very small. Because some of the most important errors are reduced by laser-cooling, we should be able to improve upon the current bound by two orders of magnitude.
Thus far, we have completed a magnetically shielded atom trap to observe and study the magnetic resonance transitions in laser cooled Cs atoms, as shown above. We have successfully laser cooled and trapped 107 Cs atoms in the trap, and observed the magnetic resonance transitions. We are currently designing and building a second-generation trap, which will incorporate improved magnetic shielding, electric field plates, and optical dipole force trapping. New measurements of the electron edm will begin shortly after the apparatus is completed. If we find such an edm, it would provide unambiguous evidence for new physics which is not included in the standard model!
Another interesting possibility which we are pursuing is that precision atomic spectroscopy experiments could be carried out in the microgravity environment of space. The microgravity environment would offer compelling advantages for such experiments. For earth-bound experiments, the maximum perturbation-free interaction time for untrapped atoms is limited by the time for the atoms to fall to the bottom of the experimental chamber due to the acceleration of gravity. Trapped atoms can provide longer interactions times, but at the expense of perturbations to the atomic level structure introduced by the trapping forces. Atoms in microgravity could be untrapped, or could be trapped with exceedingly feeble forces and with minimal perturbations. In either case, the spectroscopic accuracy which could be achieved could be dramatically increased. We are presently exploring trap designs which might be used in microgravity.